One of the most difficult processes in manufacturing pigmented systems is the process of dispersing pigments and trying to prevent from flocculation occurring. Flocculation is the recombination of dispersed pigments that are not stabilized in dispersions. In water-based systems, the problems are exacerbated due to the high surface tension of water which makes the wetting of low surface energy pigments difficult, especially for organic pigments. Inorganic pigments like titanium dioxide are relatively easy to disperse in water-based systems and dispersants with anionic properties are usually the products of choice.
However, as mentioned organic pigments have low surface energies but also have high surface areas and more complex dispersants are required.
There are generally 3 steps in the process of dispersing pigments:
• Wetting • Dispersion (grinding) • Stabilization
Before we discuss the 3 important steps of dispersion of pigments, we need some definitions of pigment particles.
Primary particles are single (or double) crystals and are the smallest particles obtained during pigment synthesis and their simple shapes can vary between cubic, rhomboidal etc.
Aggregates are rigid clusters of primary particles joined by crystal faces. Due to the high adhesion forces, dispersion does not cause aggregates to separate into primary particles.
Agglomerates are clusters of primary particles or aggregates that are loosely joined by crystal edges due to the action of van der Waals or Coulombic forces. These can be broken down, so the entire process of pigment dispersion is to divide agglomerates into primary particles and aggregates. In practice, large agglomerates usually end up being divided into smaller agglomerates.
1) Wetting is the first step of the dispersion process in which air and moisture are displaced from the pigment surface by the dispersing agent. This is done through preferential adsorption. The efficiency of wetting depends primarily on the surface tension properties between the pigment and the vehicle, as well as the viscosity of the resultant mix. The air must be fully removed and the pigment surrounded by the liquid medium.
The wetting of a solid can be described by the Young equation:
ƳsG = ƴSL + ƴLG . cos ΘC
ƴsG = solid surface free energy
ƴsl = solid/liquid interfacial free energy
ƴLG = Surface tension
Θc = contact angle of solid/liquid
To simplify the equation, if you say that spontaneous wetting occurs then Θ becomes zero and the equation becomes:
ƳSG – ƴSL = ƴLG
The action of wetting can cause surface tension to be lowered.
This means that a liquid with low surface tension will wet the pigment surface better than a high surface tension liquid so that a dispersant that acts as a wetting agent in this first step will lower the surface tension.

2) Dispersion (grinding) stage is where the primary purpose is to break down pigment aggregates and agglomerates to their optimum particulate size by milling machines or in the case of inorganic pigments the use of a high-speed mixer using a saw tooth blade. The dispersant additive speeds up the wetting of the newly created surfaces and minimizes the pigment – pigment interaction to produce a lower viscosity dispersion. During this process, the pigment particles are stabilized against flocculation and without this stabilization, the primary particles would re-agglomerate releasing the energy that was introduced into the dispersion by the grinding process.
The equation which shows the relationship between the necessary work for grinding and the breaking of agglomerates is shown below:
dW = ϒ x dA
W = work required
ϒ = Surface tension
A = Surface area
Dispersants reduce the surface tension, and from the above equation, the work needed to disperse the pigment is less. This means that the grinding time is reduced.
3) Stabilization is the most critical stage of the process and the dispersant needs to have good wetting and dispersing properties to be able, over a period of time, to keep the particles separated. If this does not happen then the particles can re-form to form flocculates. Flocculation is due to the London - Van der Waal attractive forces occurring between the particles. These forces are only effective over very small distances. In this instance, Brownian molecular motion causes particles to collide many times and creates flocculation.
The dispersant which anchors onto the pigment surface helps to repel the attractive forces between the pigment particles and stops the formation of agglomerates. This requires the dispersant to have groups which interact strongly with the pigment surface by a variety of bonding mechanisms. This stabilization of the pigment ensures that complete wetting and separation of the particles has been reached, and that the pigment particles are homogeneously distributed in the medium.
If the dispersion has not been stabilized, flocculation may occur as a result of clumping together of the pigment particles. Flocculation is generally a reversible process. Flocculates typically break down when shear is applied and will form again when the shear is removed. If the pigment dispersion is not stabilized by the resin component in the dispersion, then the use of surfactants or dispersants can be considered
Electrostatic stabilisation - this occurs when particles have the same electrical charge and hence cause repulsion between the particles. What generally happens is stabilisation through an electrical double layer, in which each layer has an equal charge and when two particles come together, their respective charged double layers overlap and repulsion occurs.
The double-layer concept

When suspensions of electrostatically charged particles are placed into an electric field of field strength E, they will migrate to the oppositely charged electrode with the velocity n. The quotient of n/E is called the is determined by the sum of the van-der Waals attractive and the electrical double layer repulsive forces. The attractive and repulsive forces depend on the interparticle separation. If the double layer is stronger, repulsion is the main force and the d ispersion is stable. However, if the double layer is affected by say the addition of salts, then the attractive forces are the main factor and the dispersion is broken up. The Schulze-Hardy law describes the influence of ionic charges on their flocculating power:
For negatively charged dispersions with increasing cationic charge the flocculating power increases: Na+ < Ca2+ < Al3+ or Fe3+
For positively charged dispersions with increasing anionic charge the flocculating power increases: Cl- < SO42- < PO43-
The stability of electrostatic interactions can be measured by their zeta potential (ζ) Zeta potential is the scientific term for electrokinetic potential and is measured in volts (V) or millivolts (mV).
Zeta potential (ζ)
In basic terms it is the potential difference between the dispersion medium and the stationary layer of the fluid attached to the dispersed particle. As the zeta potential nears zero the agglomeration of particles increases. It can be assessed by electrophoretic measurements.
When suspensions of electrostatically charged particles are placed into an electric field of field strength E, they will migrate to the oppositely charged electrode with the velocity n. Thequotient of n/E is called the electrophoretic mobility µ. The electrophoretic mobility is connected to the zeta potential ζ via the viscosity η of the liquid and its dielectric constant ε. Depending upon the relationship between the thickness of the double layer and the particle size, two cases may be distinguished. When the extension of the double layer is small in comparison to the size of the particle diameter:
ζ = µ ÷ η × ε
This is normally the case in aqueous media (Smoluchowski approximation). When the double layer is thicker than the particle diameter, the relationship is extended by a factor of 2/3 (Hückel approximation). ζ = 2 µ ÷ 3 η × ε
Traditionally, zeta potentials were determined using microelectrophoresis. The electrophoretic mobilities of the particles were measured under a microscope while an electrical field was applied. These measurements were tedious and had certain limitations. Dispersed pigment particles, for example, are mostly submicroscopic so that it is not possible to observe primary particles under the microscope. In addition, it was only possible to measure in very dilute suspensions.
Stability behaviour in relation to Zeta potential
Zeta Potential (mV) | Stability |
0 - 10 | Flocculation |
10 - 30 | Reasonable stability |
30 - 40 | Moderate stability |
40 - 60 | Good stability |
>61 | Excellent stability |
>61 | Excellent stability |
Electrostatic stabilization works for water-based systems but for solvent based and UV based systems steric stabilization plays a key role in preventing particle flocculation. This type of stabilization also occurs in water-based systems.
Steric stabilization - In this type of stabilisation polymer molecules is the generally preferred choice. They have two key features; one is a pigment affinic (anchor) group and a second binder compatible group. The anchor group attaches itself to the pigment surface and ensures strong adsorption of the dispersing additive. The binder compatible group reaches out into the solvent / UV monomer binder. Together these two features create a stabilization effect on the system.
As this process relates to energy the Gibbs – Helmholtz equation is useful for understanding how this process works.
In its simplest form the equation is:
G = H – T.S
G = Gibbs free energy
H = Enthalpy of the system
T = Temperature
S = Entropy of the system
Taking the temperature to be constant then you can say:
ΔG = ΔH – T. ΔS
If the pigment particles get too close to each other their mobility is reduced and the entropy is lowered. To balance the entropy loss the pigment particles, move away.
In the above equation, flocculation is prevented by having a positive value for ΔG.
To be effective as a polymer dispersant the additive must be highly solvated as possible and be highly compatible with the binder solution. If this is not the case then flocculation is possible. Steric stabilization is useful for both water based and non-water-based systems.
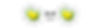
Electrosteric stabilization - Is a combination of electrostatic and steric stabilization. Specifically, this type of stabilization features adsorbed polymers like in steric stabilization, however the adsorbed polymers have non-negligible electrostatic charge. Therefore, we get significant double-layer repulsion.
Limits of stabilization - There are instances when the stabilization is compromised. This can happen at high temperatures where the primary particles move closer to each other within the range of attractive forces and the pigment flocculates. In most cases these flocculated particles can be easily separated by the application of low shear forces. One of the most common problems in the coatings market is pigment shock or solvent shock.
In pigment shock the issue is caused by adding too quickly film formers/ binders to the newly dispersed millbases in the let-down stage. The solvent diffuses out of the paste phase – low binder content into the phase which is high in binder content, to balance the concentration. This however causes the paste phase to dry out and the pigment particles to flocculate. Pigment shock is only reversible when the polymer layer adsorbed on the surface of aggregates and primary particles in the mill base ensures sufficient stabilisation for the conglomeration to behave as a form of flocculation the risk of shock can be reduced if the concentration of the film former chosen in the material to be dispersed is as high as possible and if the concentrated film former solution is added very slowly during this phase but also stirring vigorously.
Solvent shock is a similar process in that the solvent diffuses into the pigment paste, which is now richer in binder and thus reduces the stabilizing effect of the film former molecules.
Solvent shock is the occurrence of flocculation due to inaccurate solvent application rates during the production of coatings. The solvent molecules, rapidly penetrating the mill base, strip the pigment particles of their stabilising polymer layer. Pigment reagglomeration ensues and nullifies the dispersion effect This change is therefore largely irreversible. Carbon blacks and some finely-divided organic pigments are particularly sensitive to shock.
Types of dispersants:
Low molecular weight products - The original dispersing additives for pigments have been around for many years and consist of both hydrophilic and lipophilic groups. These conventional dispersants (surfactant type) tend to have molecular weights of < 2000g/mol and are characterised by their hydrophilic group, cationic, non-ionic, etc. These conventional low molecular weight polymers tend to be used for inorganic pigments and extenders / fillers. The mode of action is that the polar head of the dispersant forms hydrogen bonds with the pigments causing the particles to keep separated and stabilize the system. As these dispersants have low molecular weight, they will not give sufficient steric hindrance. These types of dispersants are good for the inorganic pigments especially the metal oxide types as they contain positive metal ions and negative oxide ions. However due to the action of these low molecular weight dispersants they are not useful for organic and black pigments.
Typical Lankem low molecular weight dispersants:
Type | Dispersant |
Anionic | |
Nonionic | |
Amphoteric |
These low molecular weight dispersants can lower the interfacial tension between the pigment and the binder solution. They act as surface active agents because they have two contrasting groups relating to solubility or polarity. For water-based systems the polar group is a hydrophilic group and the non-polar is a hydrophobic or lipophilic group. In non-water-based systems the polar group is called the oleophobic group and the non-polar one is the oleophilic group.
With these lower molecular weight dispersants their effectiveness is related to the absorption of the polar group onto the surface of the pigment and these anchoring groups can be amino, carboxylic, sulphonic, phosphoric acids and their salts and others.
The surfactant nature of these dispersants can cause certain defects like foaming, problems with intercoat adhesion and water sensitivity.
High molecular weight (polymeric) dispersants - These types of dispersants work by steric stabilisation due to their high molecular weight. Also, they have several types of functional groups which have an affinity for the pigment surface. Due to the greater molecular weights of these dispersants, they are attached to several sites on the pigment surface and hence form very stable adsorption layers. Optimum stabilization is achieved with excellent compatibility with the binder giving a well solvated polymer chain. If, however there is only one functional group per molecule of dispersant, then this leads to flocculation. As there are a few pigment types different functional groups are required in the dispersant for optimum stabilization.
Many inorganic pigments have high surface polarities due to their ionic nature and the dispersants are easily adsorbed onto the surface, for example titanium dioxide can be slightly basic and hence interaction with acid groups on dispersants is very strong.
Carbon black is one pigment that is different in that its surface area is many times that of organic or inorganic pigments. The surface area is highly acidic and hence nitrogen containing dispersants are some of the best types for excellent stabilization. The typical groups on the surface of carbon black are oxygen containing groups like carboxyl, quinones, phenol, ketones etc.
Carbon black is one pigment that is different in that its surface area is many times that of organic or inorganic pigments. The surface area is highly acidic and hence nitrogen containingdispersants are some of the best types for excellent stabilization. The typical groups on the surface of carbon black are oxygen containing groups like carboxyl, quinones, phenol, ketones etc.
Traditional types of dispersants
1) Typical comb type dispersing agent
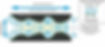
2) Typical Hyperdispersant dispersing agent - Example: Lansperse UV51

The new BioLoop dispersing agents
3) Straight BioLoop - Example: Lansperse BIO868

4) BioLoop with tails and loops - Example: Lansperse LT87
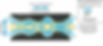